EU-TIMES
Short overview
TIMES is a modelling platform for local, national or multi-regional energy systems, which provides a technology-rich basis for estimating how energy system operations will evolve over a long term, multiple period time horizon (Loulou and Labriet, 2008) These energy system operations include the extraction of primary energy such as fossil fuels, the conversion of this primary energy into useful forms (such as electricity, hydrogen, solid heating fuels and liquid transport fuels), and the use of these fuels in a range of energy service applications (vehicular transport, building heating and cooling, and the powering of industrial manufacturing plants). In multi-region versions of the model, the trade of fuels between regions is also estimated. The TIMES framework is usually applied to the analysis of the entire energy sector, but it may also be applied to the detailed study of single sectors (e.g. the electricity and district heat sector).
The EU-TIMES model, is the multi-region, European version of TIMES, which is designed for analysing the role of energy technologies and their innovation needs for meeting European policy targets related to energy and climate change. It models technologies uptake and deployment and their interaction with the energy infrastructure in an energy systems perspective. It is a relevant tool to support impact assessment studies in the energy policy field that require quantitative modelling at an energy system level with a high technology detail. The EU-TIMES model represents the EU Member States and neighbouring countries, where each country is modelled as one region; producing projections (or scenarios) of the EU energy system showing its evolution up to 2060 under different sets of specific technology and policy assumptions and constraints.
EU-TIMES is an improved offspring of previous European energy system models developed under several EU funded projects, such as NEEDS, RES2020, REALISEGRID, REACCESS, and COMET. The EU-TIMES model has been further developed over the last years by JRC unit C.7. A list of publication is available online here.
Key features of the EU-TIMES model
Energy sectoral detail
The EU-TIMES model considers both the supply and demand sides and includes the following seven sectors: primary energy supply (including transformation); electricity generation; industry; residential; commercial; agriculture; and transport.
A simplified overview of the EU-TIMES energy sectors structure is shown in the following figure.
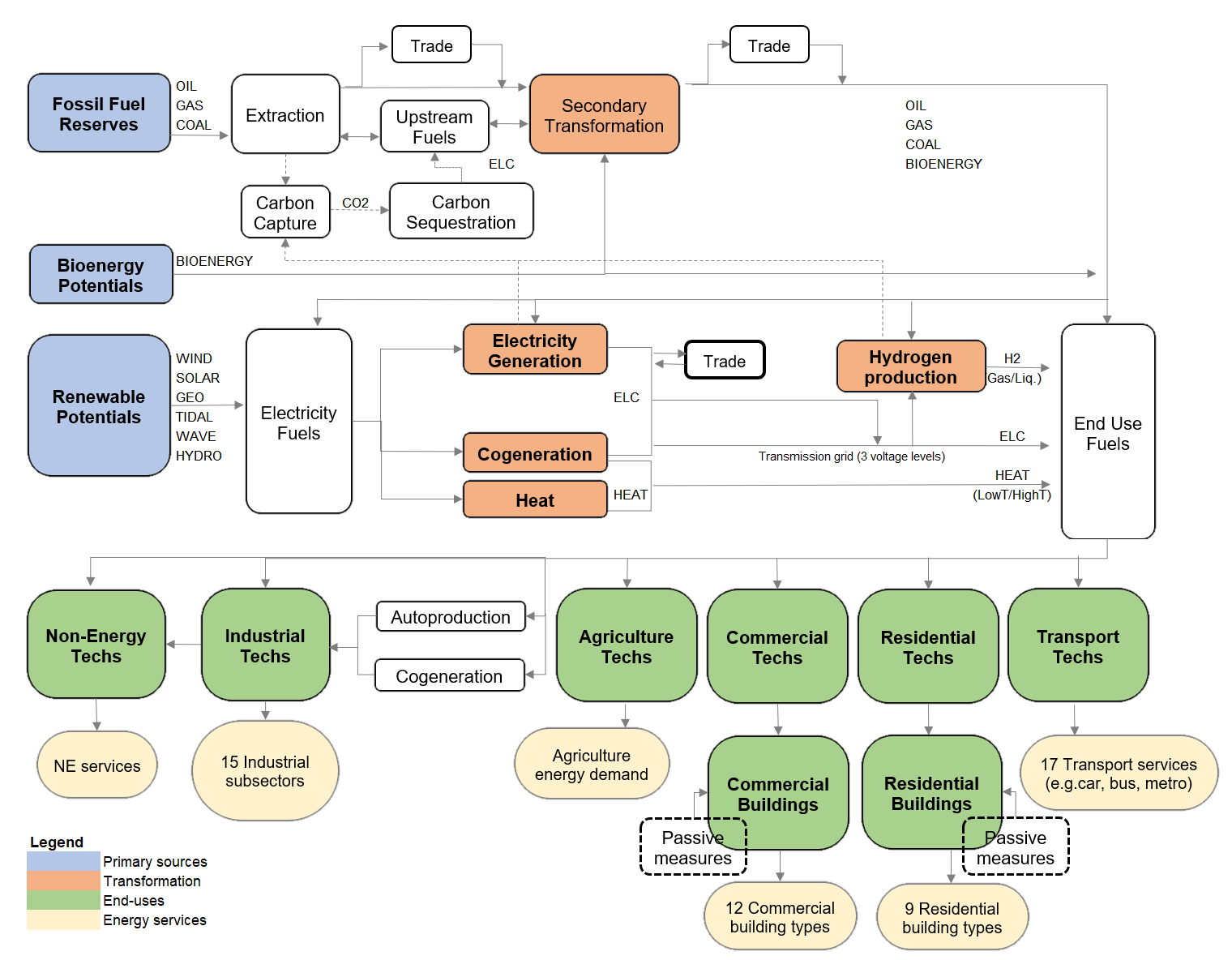
Representation of the EU-TIMES energy system for each region
Source: Own elaboration
Geographic coverage
The EU-TIMES includes in its base version 31 regions, connected by energy/emissions trade, as follows: the EU-28 (Austria, Belgium, Bulgaria, Croatia, Cyprus, Czech Republic, Germany, Denmark, Estonia, Spain, Finland, France, Greece, Hungary, Ireland, Italy, Lithuania, Luxemburg, Latvia, Malta, the Netherlands, Poland, Portugal, Romania, Sweden, Slovenia, Slovakia and the United Kingdom) and Non-EU countries (Switzerland, Iceland, Norway). The model can be optionally further expanded to the Balkans regions (Albania, Bosnia and Herzegovina, Kosovo, The Former Yugoslav Republic of Macedonia, Montenegro, and Serbia), although this configuration requires some modelling work.
Each country is represented as one single region, as shown in the following figure.
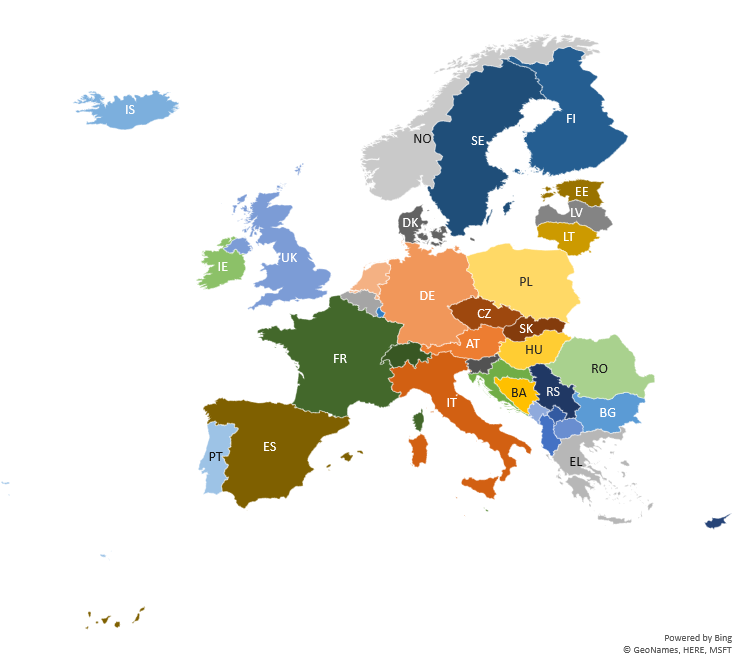
Regional representation and countries in the EU-TIMES model
Source: Own elaboration
Multi-year time periods
According to its analytical paradigm, EU-TIMES models energy dynamics over a multi period time horizon. The time horizon over which the model simulates the evolution of the energy system is divided into a user-chosen number of time-periods; which can vary depending on a number of factors, such as the scope of the analysis, model setup, computational limitations, etc.
In its current version, the EU-TIMES model runs from 2010 (base year) until 2060, with flexible number of intervals (from four to twelve). For all quantities such as installed technology levels, power plant capacities and energy and emissions flows, any annual input quantity (e.g. coal used in a power plant per year) or output quantity (e.g. electricity generated from the coal plant per year) related to a given time period applies identically to each of the years in that period.
In the framework of the project, model can run different horizons/organisation of periods (if needed/helpful).
Intra-year time periods (time slices)
In addition to the multi-year time periods described above, in the EU-TIMES there are time divisions within a year, called “time slices”, which may be defined by the user, so as to capture different weather conditions, consumer behaviours, and energy demand conditions at different times of the year. There are currently twelve time-slices representing an average of day, night and peak demand for every one of the four seasons of the year (e.g. summer day, summer night and summer peak, etc.).
Time-slices are especially important whenever the mode and cost of production of an energy carrier at different times of the year are significantly different (Loulou and Labriet, 2008). This is the case for instance when the demand for an electricity fluctuates across the year and a variety of technologies may be chosen for its production at given times of the year (such as wind power when wind resources are high, and solar photovoltaics when there is a high availability of solar radiation. In such cases, the matching of supply and demand requires that the activities of the technologies producing and consuming the electricity be tracked – and matched – in each time slice.
Emissions granularity
The model tracks the main source of greenhouse gases in the energy sector, i.e. carbon dioxide (CO2) from both combustion and industrial processing. A special module of the EU-TIMES can be enabled to allow emissions trading across the model regions.
Socioeconomic dimensions
The EU-TIMES model requires inputs concerning the degree to which energy demands, as well as demand for goods and services that result in GHG emissions, will grow over the course of the next decades in the different countries.
Key drivers to the EU-TIMES model are the socioeconomic projections of GDP growth; private consumption as a proxy for disposable income; price evolution and sector production growth, population and number of households etc.; for each country in the model. These drivers are transformed into the different final annual end-use demand projections, which are the key quantities that the EU-TIMES model must produce an energy system to satisfy. The building-related sectors (namely residential and commercial sectors) require a more detailed approach, as to generate the demands for heat, cooling and hot water, characteristics of dwellings are explicitly represented (Chiodi et al., 2017).
Across the years several sources and methodologies have been employed to generate updated EU-TIMES energy services demands. For example in Simoes et al. (2017), the materials and energy demand projections for each country are differentiated by economic sector and end-use energy service, using as a starting point historical 2005 data and macroeconomic projections from the GEM-E3 model (Russ et al., 2009) as detailed in (Simoes et al., 2013), and in line with the values considered in the EU Energy Roadmap 2050 reference scenario (EC, 2011). These projections have been further updated to align with latest socioeconomic projections in use within the European Commission (Nijs et al., 2019; Nijs et al., 2017).
Energy demand drivers and demand elasticities
In total, the EU-TIMES represents about 60 different types of energy services for the transport, residential, agricultural, commercial, industry and non-energy sectors. Some examples include number of residential and commercial dwelling stocks (thousands of dwellings), public street lighting (thousands of lighting points), industry iron & steel (millions of tonnes), transport car distance (billions of passenger kilometres) and transport road freight (billions of tonne kilometres).
The socioeconomic, population and physical drivers (e.g. physical production of industrial activities, expected numbers of new dwellings) are used as specific drivers for the growth in energy demands, associated when relevant to demand elasticities, see Loulou et al. (2016) for more details.
Once the drivers for the different energy demands represented by EU-TIMES model are selected, allocated and quantified, the construction of the reference demand scenario requires computing a set of energy service demands over the horizon.
Furthermore EU-TIMES has the capability of estimating the price-based response of these energy service demands to the changing conditions of scenarios in which mitigation occurs. For example, if the cost of energy increases as fossil fuels are replaced by renewables, then the demand for energy services would decrease. To do this, EU-TIMES makes use of another set of inputs, namely own price elasticities, applied to each model energy service. This option can be easily disabled, assuming in that case energy service demands to be inelastic.
Calibration of the model
The EU-TIMES model is calibrated to the year 2010 (base-year) and key commodity variables aligned with statistics in the period 2011-2014. The calibration makes use of a combination of the latest available information and statistics about the EU energy sectors, namely: energy balances from Eurostat and IEA (only for regions currently not covered by Eurostat), and detailed databases such JRC-IDEES, TRACCS, and ENTRANZE.
Mitigation/adaptation measures and technologies
EU-TIMES is a technology-rich model that represents most major fossil fuel and low-carbon technologies that are envisaged to be available (for those systems) for at least the first half of the 21st century. By simulating the substitution of low-carbon for high-carbon technologies in response to their relative costs, as well as emissions constraints and/or carbon prices, the model simulates mitigation. The typical question that EU-TIMES can address is what technologies are competitive under various policy scenarios. For emerging technologies with a small uptake, EU-TIMES can estimate what technology improvements would be needed to make these competitive (Nijs et al., 2018).
The principal energy sector mitigation options are shown in the table below, where the options that can be potentially added are marked with an asterisk. Data will be revised and updated in the framework of the Paris Reinforce project, and in accordance with the international database and feedback of local experts.
Moreover the EU-TIMES might be set to simulate impacts of a number of adaptation measures, as listed in the table below; such restrictions in water availability, changes in building envelope requirements, or increased requirements for specific energy services.
Upstreamy | |
---|---|
Synthetic fuel production | Hydrogen production |
|
|
Electricity generation | Heat generation |
|
|
Transport |
|
Road | Rail |
|
|
Air | Marine |
|
|
Buildings |
|
Heating | Lighting |
|
Efficiency |
Appliances | Cooling |
Efficiency | Electricity |
Behaviour change | Other |
|
Energy poverty (proxy) |
Industry |
|
Process heat | Machine drives |
|
|
Steam | CHP |
|
|
CCS | Other |
|
Demand response to price signals |
Agriculture |
|
Energy | Other |
Demand response to price signals |
*: option that can be potential added
Water | Heating & Cooling |
---|---|
Water use restrictions |
|
Economic rationale and model solution
According to its analytical paradigm, the EU-TIMES model computes a dynamic inter-temporal partial equilibrium for the (multi-) regional energy and emission markets, based on the maximisation of total surplus defined as the sum of surplus of the suppliers and consumers. In other words, it is assumed that the multi-regional system evolves, while maintaining intra and inter-temporal partial economic equilibrium, and always occupies the technical possibility frontier. The process of solving the model determines the optimal mix of technologies (capacity and activity) and fuels at each period, the associated emissions, the mining and “trading activities”, the quantity and prices of all commodities, all in time series from the base year to the time horizon of the model.
The most relevant model outputs are the annual stock and activity of energy supply and demand technologies for each region and period. This is accompanied by associated energy and material flows including emissions to air and fuel consumption, detailed for each energy carrier. Besides technical outputs, the associated operation and maintenance costs, the investment costs for new technologies, all energy and materials commodities prices (including for emissions if an emission cap is considered), are obtained for every time step.
EU-TIMES combines a social approach towards the time value of money and a private approach towards risk as well as the cost of financing based on the individual technologies. The EU-TIMES model uses a mix of private and social discount rates, since for the evaluation of investment decisions private discount rates are used, but for the timing of investment a social discount rate is applied. The first determines whether an investment pays off with the assumed private discount rate. The higher the (perceived) risk is, the higher the discount rate. Technologically specific discount rates are used to balance planning approaches and include risks for specific technologies. The second determines when is the best timing to do investments reflecting the time preference for consuming as well as a decreasing marginal utility of future consumption. This discount rate is applied primarily to make intertemporal decisions based on Net Present Value (Nijs et al., 2019).
Value of key parameters
The EU-TIMES model is “by paradigm” a technology rich tool where techno-economic information is assigned to each process (existing and future) of the system, and therefore many datasets/parameters can be extracted and reported.
In line with this concept EU-TIMES monitors cost evolution of a large subset of technologies from the upstream (e.g. costs of primary resources), transformation (e.g. electricity and hydrogen production), and final consumption (e.g. end-use sectors technologies; as appliances, cars, etc.).
Given the European context and its central focus on boosting decarbonisation, renewables and energy efficiency; the model, since its origins, had a specific focus on low carbon technologies, in particular the one identified by the EU SET Plan (Strategic Energy Technology Plan). Most recent model updates and analyses on technology have focused on improving knowledge on hydrogen and power to liquid technologies (Blanco et al., 2018; Kanellopoulos et al., 2019 and Sgobbi et al., 2016), Heating & Cooling (Chiodi et al., 2017 and Nijs et al., 2017), electromobility (Thiel et al., 2016), retrofit measures in buildings (Chiodi et al., 2017), electricity storage (Nijs et al., 2014) and other key low carbon technologies (Nijs et al., 2018) and (Simoes et al., 2017)). Datasets with renewable energy potentials (Ruiz et al., 2015 and Ruiz Castello et al., 2019) have also been made publicly available.
In the framework of the Paris Reinforce project, the following key information can be considered as the parameters to monitor, discuss and evaluate as to guarantee a successful integration with the other tools and analyses:
- - Renewable energy and CCS costs/potentials.
- - Quantities and/or prices of trade for main energy commodities.
- - Under construction/planned/possible energy projects/infrastructures.
- - Burden/contribution of Member States to the EU Energy Union objectives and other specific policies.
- - Technology costs and characteristics, in particular for key end-use sectors.
Cost and policy parameters can be revised and updated, following the feedback of national experts (stakeholder engagement), the comparative assessment with other modelling experiences, and the discussion with the partners (modellers).
Policy questions and SDGs
Key policies that can be addressed
As for all TIMES-based models, EU-TIMES can consider policies that affect either the entire energy system, sectors, group of technologies/commodities, or single technologies/commodities. For example, the following policies can be implemented:
- Carbon emissions constraint or a carbon price (imposed as a tax) in each region that it represents, or alternatively all regions simultaneously.
- Subsidies/capital grants on specific sets technologies (e.g. on solar PV).
- Constraints on the availability of particular technologies (e.g. “no nuclear”, variable renewables accounting for no more than 50% of electricity generation).
- Constraints on the growth rates of particular technologies (e.g. carbon capture and storage power generation capacity cannot grow at more than 20% per year).
- Inter-regional emissions trading (such as the EU Emissions Trading System - EU ETS).
- Technology standards (e.g. efficiency labelling or ban of specific technologies).
For EU-TIMES the most commonly applied policies include:
- Feed-in Tariffs and green certificates on renewable technologies.
- Renewable targets in gross final energy consumption (e.g. in line with EU Renewable Energy Directive RED).
- Emission targets (e.g. the EU Effort Sharing Regulation - ESR).
Furthermore, the structure of the EU-TIMES may allow (although some modelling expansion is required) the exploration of energy security concepts, with the possible analysis of scenario variants and policies related to criticalities across sensitive corridor hubs (i.e. EU REACCESS project), and security of supply (Chiodi et al., 2016).
The following table provides additional information about the capability of the model to represent policies and measures across the five key energy and climate dimensions, as defined by the governance of the EU energy union.
PaMs (by dimension) | EU-TIMES |
---|---|
Decarbonisation | High capability (several mitigation options, regulatory and economic measures, etc.) |
Energy Efficiency | High capability (several energy efficiency options, regulatory and economic measures, etc.) |
Energy Security | Medium/High capability (key focus of the previous European project REACCESS, which used the EU-TIMES in conjunction with ETSAP-TIAM model) |
Internal energy markets | Medium capability (electrification, hydrogenation, power-to-gas/liquid, interconnectivity, etc.) |
Research, Innovation and competitiveness | Low-Medium capability (implicit and/or ex-post) |
Implications for other SDGs
Apart from the above-mentioned dimensions, a number of Sustainable Development Goals (SDGs) can be taken into consideration and investigated making use of the EU-TIMES model, as shown below.
SDGs | EU-TIMES |
---|---|
§7. Affordable and clean energy (e.g., traditional biomass use, %renewable energy) | Full. Full assessment of cost-effectiveness of RES within a specific storyline. |
§8. Decent work & economic growth (e.g., impact on GDPpc, jobs) | Partial (requires further developments). The implementation of an optional TIMES plugin (currently not implemented), the macro module, may allow the estimation of GDP losses due to delivery of Paris Agreement climate targets. |
§9. Industry, innovation & infrastructure (e.g., R&D investments) | Partial. Limited to the quantification of the energy-related system costs and investment needs required to deliver energy and climate policies. |
§11: Sustainable Cities & Communities (e.g., PMs from city transport and buildings) | Limited. Although EU-TIMES does not have a geographical representation of cities and communities (i.e. each region is represented as a single node), It explicitly represents the building stock by building type (i.e. detached, semidetached and flat), by country (i.e. 37 countries) and by construction period (i.e. six periods). These outputs might inform more detailed out-of-model analyses on Sustainable Cities & Communities |
§12: Responsible production & consumption (e.g., % recycled waste, embedded emissions) | Limited. Limited to energy (and water) uses and production. |
§15: Life on land (e.g., land use for forests, rate of land use change) | Limited/Simplified. Afforestation measures can be considered; RES potential/exploitation and investment decisions (e.g. energy infrastructures) can be subject to land-specific constraints (natural and regulatory). |
Recent publications using the EU-TIMES model
Paper | Topic | Key findings |
---|---|---|
Kanellopoulos et al., 2019 | The potential role of H2 production in a sustainable future power system | The operation of a future highly decarbonised (95% CO2 emissions reduction vs 1990) power system, as defined with EU-TIMES in 2050, is analysed with the METIS model. Under the assumption of adequate competition between the electrolyser operators the resulting prices could, in most EU Member States, arrive at a sustainable equilibrium. |
Nijs et al., 2019 | Deployment scenarios for low carbon energy technologies | This report provides an outlook for deployment of a set of low carbon energy technologies, as well as background on how EU-TIMES baseline and decarbonisation scenarios are derived. The analysis shows the different role of technologies – such nuclear, CCS, hydrogen, wind, solar, ocean energy, geothermal, etc. – under two key underpinning scenarios: i) a world where Member States uses all technology options to decarbonise (including CCS and nuclear), and ii) a pro renewables world, where decarbonisation is mainly driven by renewable resources. |
Paardekooper, et al., 2018 | Heat Roadmap Europe. Quantifying the Impact of Low-carbon Heating and Cooling Roadmaps | The Heat Roadmap Europe provides scientific evidence required to effectively support the decarbonisation of the heating and cooling sector in Europe and democratise the debate about the sector. The report indicates that the EU should focus on implementing change and enabling markets for existing technologies and infrastructures in order to take advantage of the benefits of energy efficiency in a broader sense and for the heating and cooling sector specifically. |
Simoes et al., 2017 | Comparing policy routes for low-carbon power technology deployment in EU – an energy system analysis | he EU-TIMES model is used to support energy technology R&D design by analysing power technologies deployment till 2050 to different decarbonisation exogenous policy routes. Paper concludes that R&D can be tailored to depending on how sensitive technologies are to the policy routes. R&D priority should be given to those technologies that are in any case deployed rapidly across the modelled time horizon (as PV) as this could significantly reduce the energy system costs, but also to those that are deployed up to their maximum technical potentials and that are typically less sensitive to exogenous policy routes (as hydro and geothermal). For these ‘no regret’ technologies, R&D efforts could be mainly directed to increase their technical potential for implementation. For yet 'sensitive' to exogenous policy routes technologies (as CSP and marine), efforts should be assigned to improving their techno-economic characteristics such as capacity factors or associated costs. |
Sgobbi et al., 2016 | How far away is hydrogen? Its role in the medium and long-term decarbonisation of the European energy system | The EU-TIMES model is used to assess the role of hydrogen in a future decarbonised Europe under key climate scenarios. Results indicate that hydrogen could become a viable option already in 2030 e however, a long-term CO2 cap is needed to sustain the transition. |
References
Chiodi, A., De Miglio, R., Gargiulo, M., Kanudia, A., Nus, W., Politis, S., Ruiz Castello, P., and Zucker, A. (2017). EU-TIMES 2017 Upgrade: Buildings and heating & cooling technologies. JRC Technical Reports, JRC - Joint Reserch Center. doi:10.2760/602564
Simoes, S., Nijs, W., Ruiz, P., Sgobbi, A., & Thiel, C. (2017). Comparing policy routes for low-carbon power technology deployment in EU – an energy system analysis. Energy Policy, 101, 353-365. doi:10.1016/j.enpol.2016.10.006
Russ, P., Ciscar, J.-C., Saveyn, B., Soria, A., Szabo, L., Ierland, T. V., Van Regemorter, D. and Virdis, R. (2009). Economic Assessment of Post-2012 Global Climate Policies - Analysis of Greenhouse Gas Emission Reduction Scenarios with the POLESand GEM-E3 models. Tech. rep., JRC - Joint Research Center. doi:10.2791/70332
Simoes, S., Nijs, W., Ruiz, P., Sgobbi, A., Radu, D., Bolat, P., Thiel, C. and Peteves, S. (2013). The EU-TIMES - Assessing the long-term role of the SET Plan Energy technologie. JRC Scientific and Policy Reports, JRC - Joint Research Center. doi:10.2790/97596
Nijs, W., Ruiz Castello, P., & Hidalgo Gonzalez, I. (2017). Baseline scenario of the total energy system up to 2050. EU-TIMES model outputs for the 14 MS and the EU. Deliverable 5.2: Business-as-usual reference scenarios. Tech. rep., Heat Roadmap Europe.
Nijs, W., Hidalgo González, I., & Paardekooper, S. (2018). EU-TIMES and EnergyPLAN comparison. Deliverable 6.3: Methodology report for comparing the EU-TIMES and EnergyPLAN scenarios. Tech. rep., Heat Roadmap Europe.
Nijs, W., Ruiz Castello, P., Tarvydas, D., Tsiropoulos, I., & Zucker, A. (2019). Deployment scenarios for low carbon energy technologies - Deliverable D.4.7 for the Low Carbon Energy Observatory (LCEO). JRC Science for Policy Report, JRC - Joint Research Center. doi:10.2760/249336
Blanco, H., Nijs, W., Ruf, J., & Faaij, A. (2018). Potential for hydrogen and Power-to-Liquid in a low-carbon EU energy system using cost optimization. Applied Energy, 232, 617-639. doi:10.1016/j.apenergy.2018.09.216
Kanellopoulos, K., & Blanco Reano, H. (2019). The potential role of H2 production in a sustainable future power system - An analysis with METIS of a decarbonised system powered by renewables in 2050. JRC Technical Reports, JRC - Joint Research Center. doi:10.2760/540707
Loulou, R., Goldstein, G., Kanudia, A., Lettila, A., & Remme, U. (2016). Documentation for the TIMES Model - Part I. Tech. rep., IEA-ETSAP.
Thiel, C., Drossinos, Y., Krause, J., Harrison, G., Gkatzoflias, D., & Donati, A. V. (2016). Modelling Electro-mobility: An Integrated Modelling Platform for Assessing European Policies. Transportation Research Procedia, 14, 2544-2553. doi:10.1016/j.trpro.2016.05.341
Nijs, W., Simoes, S., Ruiz, P., Sgobbi, A., & Thiel, C. (2014). Assessing the role of electricity storage in EU28 until 2050. 11th International Conference on the European Energy Market (EEM14). IEEE. doi:10.1109/eem.2014.6861273
Ruiz, P., Sgobbi, A., Nijs, W., Thiel, C., Longa, F. D., Kober, T., Elbersen, B. and Hengeveld, G. (2015). The EU-TIMES model - Bioenergy potentials for EU and neighbouring countries. Tech. rep., JRC - Joint Research Center. doi:10.2790/39014
Ruiz Castello, P., Nijs, W., Tarvydas, D., Sgobbi, A., Zucker, A., Pilli, R., Camia, A., Thiel, C., Hoyer-Klick, C., Dalla Longa, F., Kober, T., Badger, J., Volker, P., Elbersen, B., Brosowki, A., Thrän, D. and Jonsson, K. (2019). ENSPRESO - an open data, EU-28 wide, transparent and coherent database of wind, solar and biomass energy potentials. Tech. rep., JRC - Joint Research Center.
Chiodi, A., Gargiulo, M., Gracceva, F., De Miglio, R., Spisto, A., Costescu, A., & Giaccaria, S. (2016). Unconventional oil and gas resources in future energy markets: A modelling analysis of the economic impacts on global energy markets and implication for Europe. JRC Science for Policy Report, JRC - Joint Research Center. doi:10.2760/34568
Paardekooper, S., Lund, R. S., Mathiesen, B. V., Chang, M., Petersen, U. R., Grundahl, L., David, A., Dahlbæk, J. and Kapetanakis, I. A., Lund, H., Bertelsen, N., Hansen, K., Drysdale, D. W. and Persson, U. (2018). Quantifying the Impact of Low-Carbon Heating and Cooling Roadmaps. Tech. rep., Aalborg university.
Sgobbi, A., Nijs, W., Miglio, R. D., Chiodi, A., Gargiulo, M., & Thiel, C. (2016). How far away is hydrogen? Its role in the medium and long-term decarbonisation of the European energy system. International Journal of Hydrogen Energy, 41, 19-35. doi:10.1016/j.ijhydene.2015.09.004